BOLIK Stéphanie1,2, SCHLAICH Alexander3,4, MUKHINA Tetiana5, AMATO Alberto1, BASTIEN Olivier1, SCHNECK Emanuel5, DEMÉ Bruno2, and JOUHET Juliette1.
1 Laboratoire Physiologie Cellulaire et Végétale, Univ. Grenoble Alpes, CNRS, CEA, INRAE, IRIG, Grenoble, France.
2 Large Scale Structures group, Institut Laue-Langevin, 38000, Grenoble, France.
3 Institute for Computational Physics, Universität Stuttgart, Stuttgart, Germany
4 Stuttgart Center for Simulation Science (SimTech), Universität Stuttgart, Stuttgart, Germany
5 Institute for Condensed Matter Physics, TU Darmstadt, Darmstadt, Germany
Our work gives a possible explanation for an intriguing question that is unanswered for more than 30 years, since the discovery of betaine lipids: why seed plants lost their capacity to synthesize them if betaine lipids are a good substitute for phospholipids during phosphate starvation?
Indeed, phosphate is a vital element for plant and algae growth, yield, and survival, but in most environments, soil or water, it is poorly available. Under phosphate deficiency, plants and algae activate a range of adaptive responses including transcriptomic, metabolic, and developmental changes. One of their strategy is to use their phospholipids as a phosphate reservoir. Hence in response to phosphate starvation, phospholipids are degraded, to release phosphate for vital cellular processes, and the lost phospholipids are replaced with non-phosphorous glycerolipids. The latter point has been well documented in plants (Jouhet et al, 2004; Andersson et al, 2005), where glycolipids replace phospholipids in membranes. In bacteria, fungi, or microalgae, cells become also depleted of membrane phospholipids but phospholipids seem to be replaced by betaine lipids (Abida et al, 2015; Muhlroth et al, 2017; Riekhof et al, 2005a; Senik et al, 2015).
Betaine lipids are assumed to be analogues of the main eukaryote phosphoglycerolipid phosphatidylcholine (PC). Because betaine lipids are localized in extrachloroplastic membranes in algae (Eichenberger, 1993; Kunzler et al., 1997), and because betaine lipids have a common structural fragment with PC (Eichenberger et al., 1993; Kato et al., 1995) (Figure 1), it was speculated that these two lipid classes are interchangeable within the membrane structure, but this was never demonstrated.
In higher plants, the synthesis of betaine lipids is lost, driving plants to other strategies to cope with phosphate starvation: in extraplastidic membrane they replace PC by a plastid synthesized glycolipid, digalactosyldiacylglycerol (DGDG). However, in previous works, we showed that the physicochemical properties of PC and DGDG are not so similar (Demé et al., 2014 FASEB J; Kanduc et al., 2017 Nature Com). Therefore, in phosphate starvation, DGDG is not fully complementing PC’s structural role.
Why did seed plants lose their ability to synthesize betaine lipids and adopt a complex strategy of lipid replacement to cope with phosphate starvation if betaine lipids were a good substitute for PC?
This is the question we address in this article where we used the D16 small-angle neutron diffractometer of the ILL to perform diffraction experiments on solid-supported membrane multilayers and SANS on multilamellar vesicle solutions, combined to molecular dynamics simulations, and the phylogeny of betaine lipid synthesis. We show that betaine lipid bilayers are thicker, more rigid, and mutually more repulsive than PC bilayers (Figure 2). Furthermore, the evolution of betaine lipid biosynthesis splits between marine and freshwater organisms and does not follow the taxonomy, suggesting a different selection pressure acting on marine and freshwater environments. Altogether, our results provide an explanation for the diversity of betaine lipids observed in marine organisms and for their disappearance in seed plants.
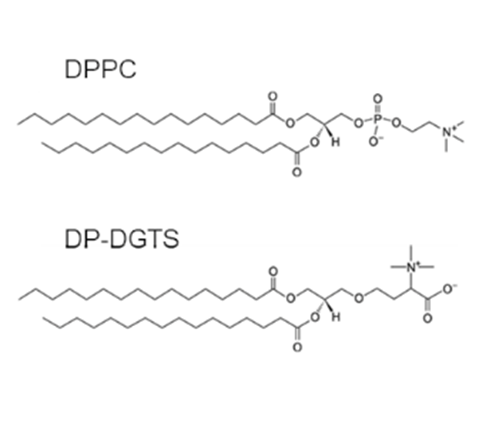
Figure 1: Lipid chemical structures of Dipalmitoylphosphatidylcholine (DPPC) and Dipalmitoylglyceryl-N,N,N-trimethyl-homoserine (DP-DGTS).
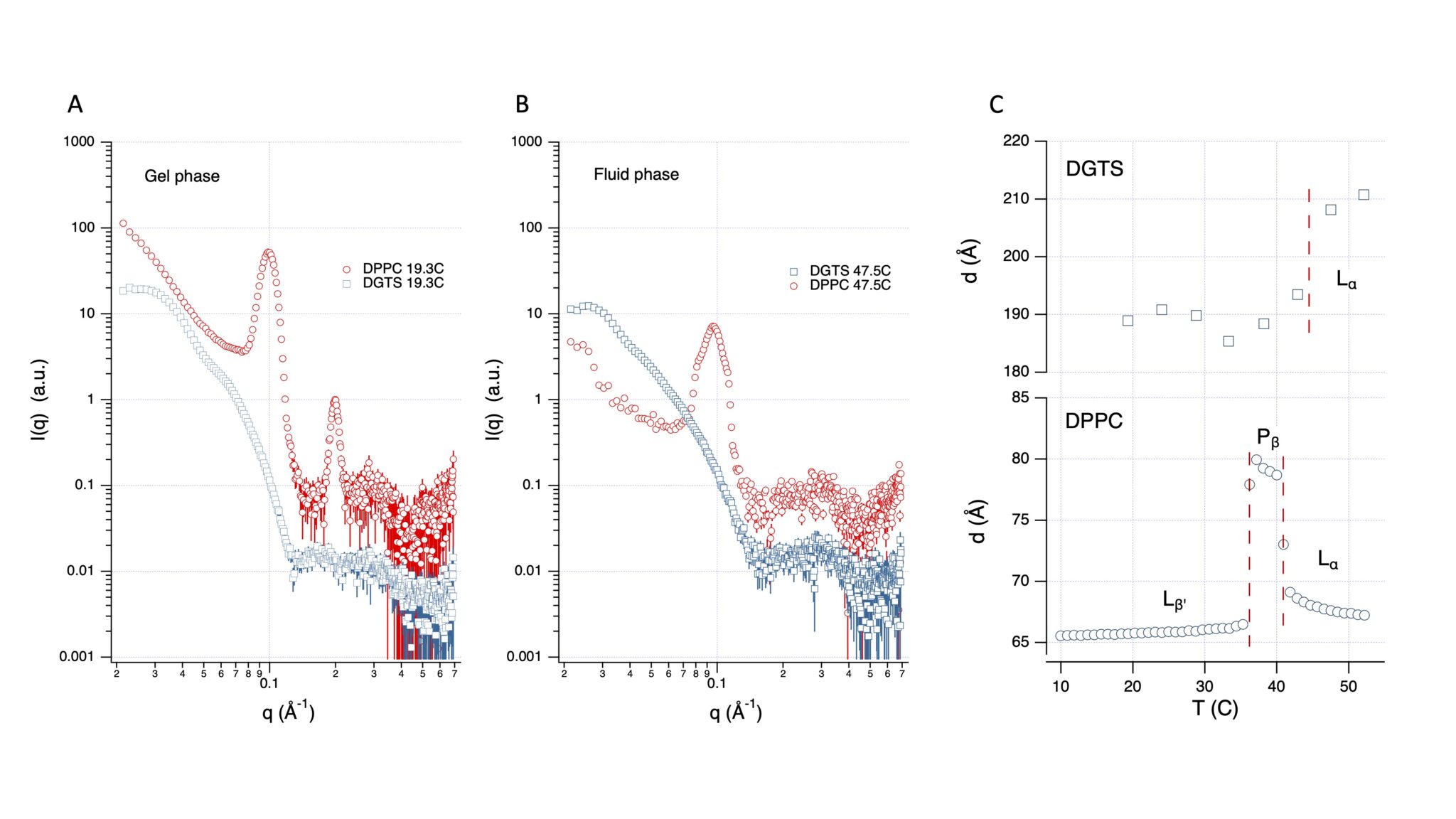
Figure 2: Periodicity of DGTS bilayers is much higher than for PC bilayers at full hydration. SANS curves (D16) of multilamellar vesicles in excess water made of DP-DGTS (blue symbols, scaled by 10) and DPPC lipids (red symbols) in gel phase A (T = 19.3°C) or in fluid phase B (T= 47.5°C). C: Lamellar period of DP-DGTS and DPPC as a function of temperature. For DPPC the two phase transition temperatures are directly deduced from the sharp period changes and represented by the vertical dashed lines. For DP-DGTS, where no sharp change is observed, the dashed line corresponds to the gel-fluid transition temperature (43.8°C) obtained by DSC. No ripple phase Pβ was detected for DP-DGTS bilayers.
References:
- Abida, H., Dolch, L.-J., Meï, C., Villanova, V., Conte, M., Block, M.A., Finazzi, G., Bastien, O., Tirichine, L., Bowler, C., Rébeillé, F., Petroutsos, D., Jouhet, J., Maréchal, E., 2015. Membrane glycerolipid remodeling triggered by nitrogen and phosphorus starvation in Phaeodactylum tricornutum. Plant Physiol 167, 118–136. https://doi.org/10.1104/pp.114.252395
- Andersson, M.X., Larsson, K.E., Tjellström, H., Liljenberg, C., Sandelius, A.S., 2005. Phosphate-limited oat. The plasma membrane and the tonoplast as major targets for phospholipid-to-glycolipid replacement and stimulation of phospholipases in the plasma membrane. J Biol Chem 280, 27578–27586. https://doi.org/10.1074/jbc.M503273200
- Demé, B., Cataye, C., Block, M.A., Maréchal, E., Jouhet, J., 2014. Contribution of galactoglycerolipids to the 3-dimensional architecture of thylakoids. The FASEB Journal 28, 3373–3383. https://doi.org/10.1096/fj.13-247395
- Eichenberger, W., Araki, S., Müller, D.G., 1993. Betaine lipids and phospholipids in brown algae. Phytochemistry, The International Journal of Plant Biochemistry 34, 1323–1333. https://doi.org/10.1016/0031-9422(91)80024-U
- Jouhet, J., Maréchal, E., Baldan, B., Bligny, R., Joyard, J., Block, M.A., 2004. Phosphate deprivation induces transfer of DGDG galactolipid from chloroplast to mitochondria. J Cell Biol 167, 863–874. https://doi.org/10.1083/jcb.200407022
- Kanduč, M., Schlaich, A., de Vries, A.H., Jouhet, J., Maréchal, E., Demé, B., Netz, R.R., Schneck, E., 2017. Tight cohesion between glycolipid membranes results from balanced water-headgroup interactions. Nat Commun 8, 14899. https://doi.org/10.1038/ncomms14899
- Kato, M., Sakai, M., Adachi, K., Ikemoto, H., Sano, H., 1996. Distribution of betaine lipids in marine algae. PHYTOCHEMISTRY 42, 1341–1345. https://doi.org/10.1016/0031-9422(96)00115-X
- Künzler, K., Eichenberger, W., Radunz, A., 1997. Intracellular localization of two betaine lipids by cell fractionation and immunomicroscopy. Z Naturforsch C J Biosci 52, 487–495.
- Mühlroth, A., Winge, P., El Assimi, A., Jouhet, J., Maréchal, E., Hohmann-Marriott, M.F., Vadstein, O., Bones, A.M., 2017. Mechanisms of Phosphorus Acquisition and Lipid Class Remodeling under P Limitation in a Marine Microalga. Plant Physiol 175, 1543–1559. https://doi.org/10.1104/pp.17.00621
- Riekhof, W.R., Naik, S., Bertrand, H., Benning, C., Voelker, D.R., 2014. Phosphate Starvation in Fungi Induces the Replacement of Phosphatidylcholine with the Phosphorus-Free Betaine Lipid Diacylglyceryl-N,N,N-Trimethylhomoserine. Eukaryotic Cell 13, 749–757. https://doi.org/10.1128/ec.00004-14
- Senik, S.V., Maloshenok, L.G., Kotlova, E.R., Shavarda, A.L., Moiseenko, K.V., Bruskin, S.A., Koroleva, O.V., Psurtseva, N.V., 2015. Diacylglyceryltrimethylhomoserine content and gene expression changes triggered by phosphate deprivation in the mycelium of the basidiomycete Flammulina velutipes. Phytochemistry 117, 34–42. https://doi.org/10.1016/j.phytochem.2015.05.021